Magnetic Fields Around Compact Objects Workshop
PI/4-405 - Bob Room
Perimeter Institute for Theoretical Physics
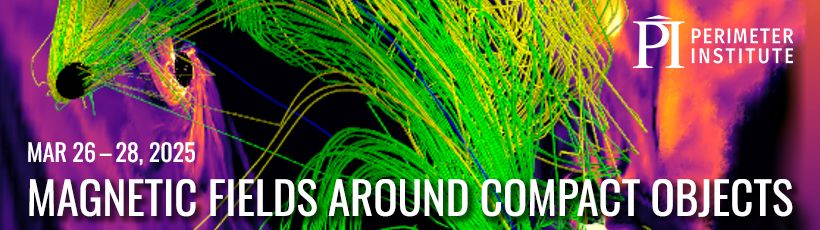
Scientific Organizers:
Luciano Combi (Perimeter Institute & U of Guelph)
Sean Ressler (CITA)
Bart Ripperda (CITA)
Luis Lehner (Perimeter Institute)
Will East (Perimeter Institute)
Gibwa Musoke (CITA)
Chris Thompson (CITA)
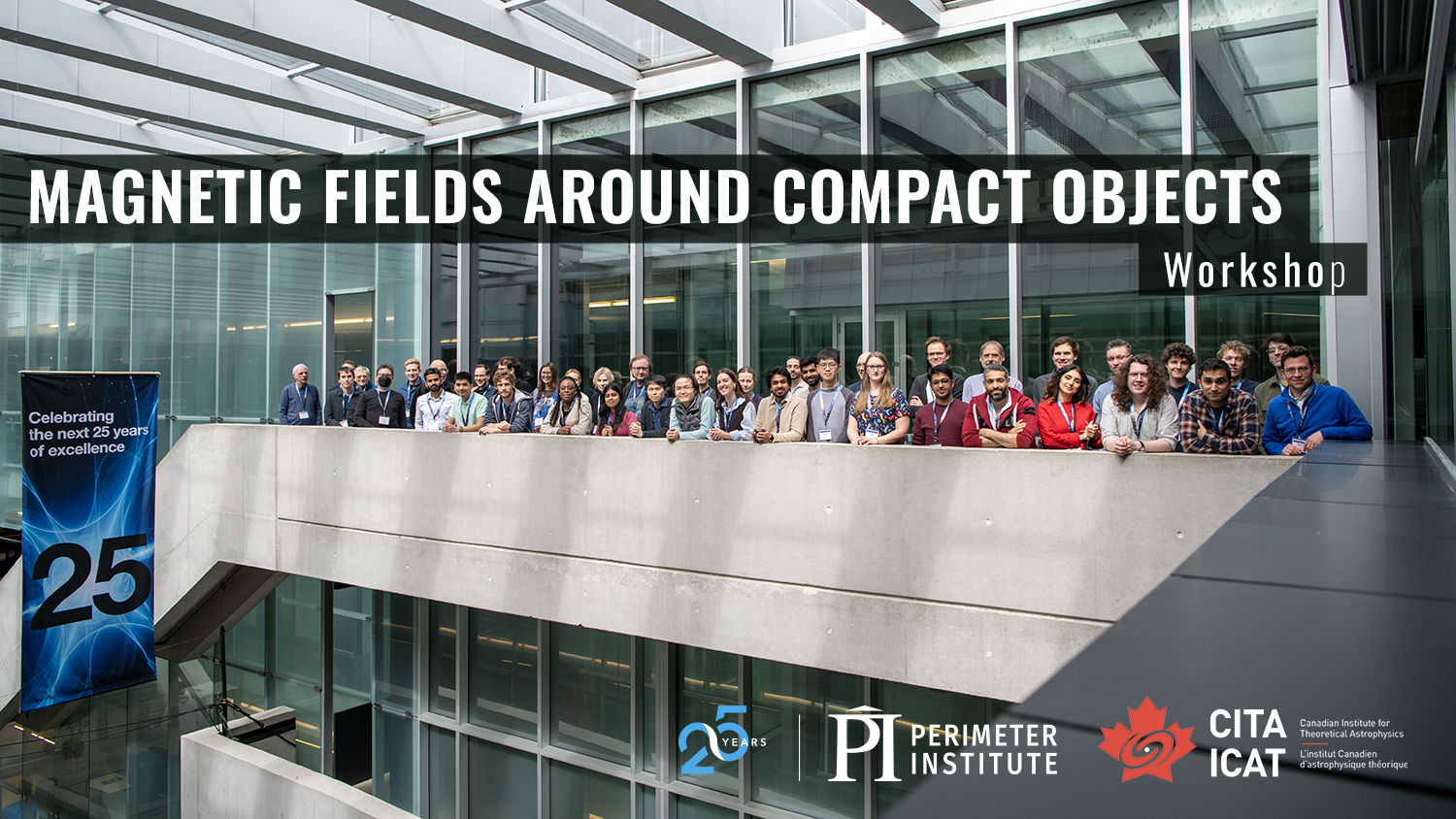
-
-
8:30 a.m.
Registration
-
1
Opening Remarks PI/4-405 - Bob Room
PI/4-405 - Bob Room
Perimeter Institute for Theoretical Physics
60- 25030072
- a51b25a5-4f4e-407f-b2c3-d044a85eea70
-
2
Snap, Crackle and Pop PI/4-405 - Bob Room
PI/4-405 - Bob Room
Perimeter Institute for Theoretical Physics
60An alternative interpretation of the EHT observations of M87, involving a strongly magnetized ergomagnetosphere, that expels essentially of the gas supplied at large radius as a jet-collimating MHD wind is described. In this case, the emitting regions are approximated as rapidly evolving flares or sparks. Six generic consequences, that can be sought in existing data, are described.
Speaker: Roger Blandford (Roger Blandford)- 25030073
- ad2f84e8-e482-42dc-8edd-684ad04585a2
-
3
Modeling Luminous Black Hole Accretion Flows with AthenaK PI/4-405 - Bob Room
PI/4-405 - Bob Room
Perimeter Institute for Theoretical Physics
60A new version of the Athena++ AMR MHD code implemented using the Kokkos library for performance-portability will be described. The code is being used for a variety of problems related to accretion onto (and merger of) compact objects. This talk will focus on results from general relativistic radiation MHD models of accretion in luminous systems such as X-ray binaries and AGN. The models allow observationally important quantities such as the radiative efficiency, variability, and kinetic feedback in winds to be measured over a range of luminosities, from sub-Eddington to highly super-Eddington. A new “cyclic-zoom” approach for modeling such flows over very long time scales will also be briefly introduced.
Speaker: James Stone (IAS)- 471d864d-f44c-48c3-82d1-c640b7714e92
- 25030074
-
4
Black Hole Jet Sheath as a Candidate for the Comptonizing Corona PI/4-405 - Bob Room
PI/4-405 - Bob Room
Perimeter Institute for Theoretical Physics
60What powers the hard, non-thermal X-rays from accreting compact objects has been a longstanding mystery. In my talk, I will address the underlying question of what energizes the particles of the Comptonizing “corona” against the strong inverse Compton (IC) cooling losses with first-principle particle-in-cell simulations of magnetic reconnection subject to IC cooling in magnetically dominated electron-positron plasmas, and in mildly-magnetized electron-ion plasmas. I will also show---using results of global resistive GRMHD simulations of accreting black holes---that the black hole jet sheath is a site of efficient electromagnetic dissipation through processes such as magnetic reconnection and turbulence. The distribution of bulk motions of the radially outflowing plasma along the jet sheath also resembles a Maxwellian distribution with an effective bulk temperature of a few 100 keV, and this could be a candidate for the Comptonizing corona.
Speaker: Dr Navin Sridhar (Stanford)- 25030131
- e6e7c60b-1f4f-4c1c-baa3-e1974b0f86d4
-
5
Hot, Retrograde Tilted MADs: Misaligned, Precessing, and Shaped by Electromagnetic Torques PI/4-405 - Bob Room
PI/4-405 - Bob Room
Perimeter Institute for Theoretical Physics
60Tilted magnetically arrested disks (MADs) around black holes—where strong magnetic fields regulate accretion and jets—exhibit striking alignment behavior dictated by black hole spin direction. Using 3D general-relativistic magnetohydrodynamic (GRMHD) simulations of tilted MADs, we find that prograde disks align via a two-stage process: an initial rapid alignment phase, ending at the flux saturation timescale, followed by a slower, spin-independent phase. In contrast, retrograde MADs remain persistently misaligned, with their inner disks precessing four times faster than weakly magnetized systems—a potential explanation for high-frequency quasi-periodic oscillations (QPOs). By analyzing magnetic and hydrodynamic torques within ideal GRMHD, we show that alignment in prograde disks is dominated by electromagnetic stresses from the magnetosphere. However, the same magnetic forces— which always act to align the disk with the black hole spin—are significantly weaker in retrograde disks, allowing opposing hydrodynamic torques to dominate. These results suggest that jets alone may not be sufficient to align MAD disks, instead highlighting the magnetosphere’s crucial role in mediating spin-disk coupling.
Speaker: Mr Sajal Gupta (University of Colorado Boulder)- 25030132
- f477a7b7-4ef7-436b-96d0-ad2a3532eada
-
10:45 a.m.
Break PI/4-405 - Bob Room
PI/4-405 - Bob Room
Perimeter Institute for Theoretical Physics
60 -
6
Workshop Talk PI/4-405 - Bob Room
PI/4-405 - Bob Room
Perimeter Institute for Theoretical Physics
60Speaker: Luciano Combi (Perimeter Institute)- b1f162f4-867b-4755-b741-1bb7efbf90d7
- 25030185
-
7
Rethinking The Black Hole Corona as an Extended, Multizone Outflow PI/4-405 - Bob Room
PI/4-405 - Bob Room
Perimeter Institute for Theoretical Physics
60Observations of luminous black holes in X-ray binaries and Seyfert galaxies show power-law emission, thought to originate from photons that inverse Compton scatter off a hot electron cloud. If the coronal electrons are heated by magnetic dissipation, i.e. reconnection or turbulence, then one might expect to observe direct synchrotron emission in the radio/mm from these electrons. However, because timing studies
constrain the X-ray emission to be within ~10 rg of the central black hole, the direct synchrotron emission from this compact volume would be strongly self-absorbed until much further away from BH. In this talk, I will question the de facto definition of the corona as a compact, X-ray-emitting region and shift instead to a paradigm where the corona encompasses multiple layers with distinct spectral components. Motivated
by highly-magnetized winds found in GRMHD simulations, I will present a model for such an extended, outflowing corona. I will discuss this model in the context of radio-quiet AGN, where recent observations have demonstrated the presence of compact mm emission.Speaker: Lia Hankla (University of Maryland, College Park)- 11784188-f94b-4aac-975c-4a98853de1a9
- 25030112
-
Lightning Talk: Lightning Talks PI/4-405 - Bob Room
PI/4-405 - Bob Room
Perimeter Institute for Theoretical Physics
60-
8
Quantifying flux rope characteristics in relativistic 3D reconnection simulations
Plasmoid-dominated magnetic reconnection is known to convert magnetic energy into heat and kinetic energy and is thought to be closely related to high-energy emission features originating near compact objects. We present preliminary results of high-resolution special-relativistic resistive magnetohydrodynamic simulations of reconnecting 3D current sheets starting from a Harris equilibrium. We focussed on identifying and quantifying flux rope structures and how the properties of produced magnetosonic waves (potentially generating winds around compact objects) rely on the underlying plasma description. We show that while the initial stage does not differ substantially from 2D results, a secondary turbulent reconnection phase can only be studied in 3D.
Speaker: Dr Jesse Vos (KU Leuven)- 6cd89ef2-ffac-40c2-a923-2d401cbdfe81
- 25030133
-
9
Understanding Magnetic Dissipation in the Magnetar Magnetosphere Regime
Magnetars produce the brightest detected outbursts in the X-ray and radio bands, offering unique opportunities to probe extreme plasma physics and exotic quantum electrodynamic processes. Magnetospheric reconnection is a suspected mechanism for generating bursts and giant flares. However, modeling the interplay between small-scale reconnection processes and the global structure of magnetar magnetospheres remains a major theoretical and computational challenge. This is due to the multi-scale nature of the problem: resolving localized reconnection while capturing the global dynamics of the star in a single numerical simulation is computationally prohibitive. Global models have primarily used force-free schemes which are unable to capture dissipation, recent advances have made global ideal magnetohydrodynamic models viable but will still lack the explicit resistivity needed to carefully study the reconnection physics. This motivates the use of local resistive relativistic magnetohydrodynamic simulations which probe the details of reconnection in the magnetospheric regime.
These local simulations allow for the quantification of magnetic energy dissipation and the role it plays in powering emission due to magnetic reconnection inaccessible to force-free models. Furthermore, the use of ideal global models motivates the careful quantification of numerical dissipation in ideal schemes.We use 1D and 2D tests to quantify the nature of magnetic dissipation in resistive and ideal, relativistic magnetohydrodynamic and force-free-electrodynamic schemes. Our tests, which are agnostic to the form of the effective numerical dissipation operator in ideal schemes, probe both Ohmic dissipation and magnetic reconnection of current structures in the highly magnetized strong guide field regime. These tests characterize exactly how well schemes commonly used to model magnetic dissipation in magnetar and pulsar magnetospheres perform compared to resistive relativistic magnetohydrodynamics. We find Ohmic dissipation in both ideal magnetohydrodynamic and force-free schemes to be subdiffuse, while producing an analogue to the Sweet-Parker regime at low resolutions and an asymptotic reconnection rate at high resolutions. The resistive force-free scheme we test is found to exactly reproduce the Ohmic diffusion and the Sweet-Parker regime, but differs from the full resistive relativistic magnetohydrodynamic result in the asymptotic regime.
Speaker: Mr Michael Grehan (University of Toronto)- 579e138d-4ed5-4e3f-8ba9-7dff1f47acce
- 25030134
-
10
Ion-synchrotron emission from reconnecting current sheets in M87
It has been proposed recently that Inverse Compton scattering of soft photons by pairs acceleration in reconnecting current sheets that form during MAD states, can be the source of the TeV emission detected in M87. In this talk I’ll argue that synchrotron emission by ions accelerated in the current sheet is expected to be the dominant source of the GeV emission observed. The analysis is based on 3D, radiative PIC simulations of magnetic reconnection in pair-ion plasma, under conditions anticipated in M87 during MAD states.
Speaker: Amir Levinson (Tel Aviv university)- 247d7ce2-07d8-4f40-a2a3-9128b9873cd2
- 25030152
-
8
-
12:30 p.m.
Lunch PI/4-405 - Bob Room
PI/4-405 - Bob Room
Perimeter Institute for Theoretical Physics
60 -
11
Colloquium: Radiation of Extreme Plasmas near the Neutron Stars and Black holes PI/4-405 - Bob Room
PI/4-405 - Bob Room
Perimeter Institute for Theoretical Physics
60Astrophysical compact objects, neutron stars, and black holes are powerful sources of non-thermal electromagnetic emission spanning many orders of magnitude in photon energy, from radio waves to multi-TeV gamma rays. Despite multiple groundbreaking observational discoveries done in recent years, our understanding of the dynamics of relativistic plasmas that produce these emission signatures remains limited. In this talk, I will describe a few successful examples of modeling the observed light coming from these remarkable astrophysical laboratories using various numerical approaches. I will focus on advances in understanding coherent radio emission of rotating neutron stars, pulsars, and multi-wavelength flares from accreting black holes.
Speaker: Alexander Philippov (University of Maryland)- 25030080
- 394c798d-f0aa-4cbc-9758-a18fa20a3f1e
-
3:00 p.m.
Break PI/4-405 - Bob Room
PI/4-405 - Bob Room
Perimeter Institute for Theoretical Physics
60 -
12
The Impact of Plasma Angular Momentum on Magnetically Arrested Flows and Relativistic Jets in Hot Accretion Flows Around Black Holes PI/4-405 - Bob Room
PI/4-405 - Bob Room
Perimeter Institute for Theoretical Physics
60In certain scenarios, the accreted angular momentum of plasma onto a black hole could be low; however, how the accretion dynamics depends on the angular momentum content of the plasma is still not fully understood. We present three-dimensional, general relativistic magnetohydrodynamic simulations of low angular momentum accretion flows around rapidly spinning black holes (with spin $a = +0.9$). The initial condition is a Fishbone-Moncrief (FM) torus threaded by a large amount of poloidal magnetic flux, where the angular velocity is a fraction $f$ of the standard value. For $f = 0$, the accretion flow becomes magnetically arrested and launches relativistic jets but only for a very short duration. After that, free-falling plasma breaks through the magnetic barrier, loading the jet with mass and destroying the jet-disk structure. Meanwhile, magnetic flux is lost via giant, asymmetrical magnetic bubbles that float away from the black hole. The accretion then exits the magnetically arrested state. For $f = 0.1$, the dimensionless magnetic flux threading the black hole oscillates quasi-periodically. The jet-disk structure shows concurrent revival and destruction while the gas efficiency at the event horizon changes accordingly. For $f \geq 0.3$, we find that the dynamical behavior of the system starts to approach that of a standard accreting FM torus. Our results thus suggest that the accreted angular momentum is an important parameter that governs the maintenance of a magnetically arrested flow and launching of relativistic jets around black holes.
Speaker: Mr Leon Chan (Unviersity of Colorado, Boulder)- 25030135
- 286d0c74-473c-4626-afbc-c41b3bb202cb
-
13
AsterX: a new open-source GPU-accelerated GRMHD code for dynamical spacetimes PI/4-405 - Bob Room
PI/4-405 - Bob Room
Perimeter Institute for Theoretical Physics
60With the ongoing transition toward exascale computing to tackle a range of open questions via numerical simulations, the development of GPU-optimized codes has become essential. In this talk, I will highlight the key features of AsterX, a novel open-source, modular, GPU-accelerated general relativistic magnetohydrodynamic (GRMHD) code for fully dynamical spacetimes in 3D Cartesian coordinates. Built for exascale applications, AsterX integrates with CarpetX, the new driver for the Einstein Toolkit, leveraging AMReX for block-structured adaptive mesh refinement (AMR). The code employs the flux-conservative Valencia formulation for GRMHD, and uses high-resolution shock capturing schemes to ensure accurate hydrodynamic modeling. Alongside discussions on the ongoing code development, I will also present the results of comprehensive 1D, 2D, and 3D GRMHD tests conducted on OLCF's Frontier supercomputer, highlighting AsterX's performance gains through subcycling in time and demonstrating its scaling efficiency across thousands of nodes.
Speaker: Dr Liwei Ji (Rochester Institute of Technology)- 25030184
- d2dac0f4-d337-42dd-add6-d3830ef3d813
-
14
Panel Discussion #1 PI/4-405 - Bob Room
PI/4-405 - Bob Room
Perimeter Institute for Theoretical Physics
60- 235e2b45-6574-4722-bc10-22d3adf87310
- 25030137
-
6:00 p.m.
Banquet PI/4-405 - Bob Room
PI/4-405 - Bob Room
Perimeter Institute for Theoretical Physics
60
-
8:30 a.m.
-
-
15
Neutron Star Mergers and AthenaK PI/4-405 - Bob Room
PI/4-405 - Bob Room
Perimeter Institute for Theoretical Physics
60In this talk, I will explore key open questions in our understanding of neutron star mergers and their multi-messenger emission, highlighting recent progress made by our group. I will then introduce some of the first applications of AthenaK to compact binary mergers and outline our plans for its future development.
Speaker: David Radice (Pennsylvania State University)- fb64d28c-4d85-4720-b762-416721348c63
- 25030086
-
16
General Relativistic Magnetohydrodynamics Simulations of Accreting Supermassive Black Hole Binaries PI/4-405 - Bob Room
PI/4-405 - Bob Room
Perimeter Institute for Theoretical Physics
60Supermassive black hole mergers represent a spectacular cosmic event with immense energy implications, emitting gravitational waves equivalent to the total light output of stars in the entire Universe within a brief timespan. These mergers play a crucial role in shaping the overall mass distribution of supermassive black holes across the cosmos. However, capturing visual evidence of these mergers remains elusive due to uncertainties surrounding the type of light emissions accompanying gravitational waves during these events. To address this challenge, novel General Relativistic Magnetohydrodynamics (GRMHD) simulations are being conducted to gain detailed insights into the astrophysical environments surrounding supermassive black hole binaries as they progress towards merger. By employing sophisticated computational techniques capable of accurately capturing the intricate dynamics of accretion within circumbinary disks and the relativistic flow of magnetized matter around each black hole, these simulations reveal the behavior of gas flows near binary systems, particularly when both black holes exhibit spin. These simulated scenarios provide critical data for predicting the electromagnetic and gravitational wave signatures produced by supermassive binary black holes, guiding future observational strategies utilizing advanced missions like LISA and other upcoming astronomical facilities. Ongoing initiatives are focused on refining computational tools to deepen our understanding of supermassive black hole behavior within binary systems and its interactions.
Speaker: Manuela Campanelli- 25030087
- a596ee91-59bb-4957-bd0d-5567d81c2f46
-
17
Long-term impact of the magnetic-field strength on the evolution and electromagnetic emission by neutron-star merger remnants PI/4-405 - Bob Room
PI/4-405 - Bob Room
Perimeter Institute for Theoretical Physics
60Numerical simulations are essential to understand the complex physics accompanying the merger of binary
systems of neutron stars. However, these simulations become computationally challenging when they have to
model the merger remnants on timescales over which secular phenomena, such as the launching of magneti-
cally driven outflows, develop. To tackle these challenges, we have recently developed a hybrid approach that
combines, via a hand-off transition, a fully general-relativistic code (FIL) with a more efficient code mak-
ing use of the conformally flat approximation (BHAC+). We here report important additional developments of
BHAC+ consisting of the inclusion of gravitational-wave radiation-reaction contributions and of higher-order
formulations of the equations of general-relativistic magnetohydrodynamics. Both improvements have allowed
us to explore scenarios that would have been computationally prohibitive otherwise. More specifically, we have
investigated the impact of the magnetic-field strength on the long-term (i.e., ∼ 200 ms) and high-resolution
(i.e., 150 m) evolutions of the “magnetar” resulting from the merger of two neutron stars with a realistic equa-
tion of state. In this way, and for sufficiently large magnetic fields, we observe the loss of differential rotation
and the generation of magnetic flares in the outer layers of the remnant. These flares, driven mostly by the
Parker instability, are responsible for intense and collimated Poynting flux outbursts and low-latitude emissions.
This novel phenomenology offers the possibility of seeking corresponding signatures from the observations of
short gamma-ray bursts and hence revealing the existence of a long-lived strongly magnetized remnant.Speaker: Dr Michail Chabanov (Rochester Institute of Technology)- 25030141
- e799dd00-1417-428d-9755-e0e92cfc159c
-
18
Magnetic field effects in binary neutron star mergers: insights from GRMHD simulations PI/4-405 - Bob Room
PI/4-405 - Bob Room
Perimeter Institute for Theoretical Physics
60Magnetic fields play a key role in shaping the dynamics and observational phenomenology of binary neutron star (BNS) mergers. In this talk, I will present results from general relativistic magnetohydrodynamic (GRMHD) simulations performed with the code GR-Athena++, exploring how different initial magnetic field configurations affect the evolution of BNS mergers. We investigated magnetic field amplification, primarily driven by the Kelvin-Helmholtz instability, the post-merger remnant and disk structure, and the characteristics of the ejected material. I will discuss how these processes impact potential electromagnetic counterparts and their detectability. Finally, I will highlight recent advancements in our numerical methods that improve the modeling of magnetized neutron star mergers, paving the way for more accurate predictions of multimessenger signals from these extreme events.
Speaker: Dr Eduardo Gutierrez (The Pennsylvania State University)- 25030142
- 749525e7-0817-4f4c-88c5-c95c20c94c61
-
10:30 a.m.
Break PI/4-405 - Bob Room
PI/4-405 - Bob Room
Perimeter Institute for Theoretical Physics
60 -
19
Dark Photon Superradiance PI/4-405 - Bob Room
PI/4-405 - Bob Room
Perimeter Institute for Theoretical Physics
60Gravitational and electromagnetic signatures of black hole superradiance are a unique probe of ultralight particles that are weakly-coupled to ordinary matter. Through the kinetic mixing with the Standard Model photon, a dark photon superradiance cloud sources a rotating visible electromagnetic field. I will describe how this leads to the production of a turbulent pair plasma, characterized by efficient magnetic reconnection, which radiates large-luminosity high-energy electromagnetic emissions. This enables multi-messenger search strategies to probe unconstrained regions of parameter space.
Speaker: Nils Peter Siemonsen- 55c694be-5f2f-4741-8f7d-5662d15ab1c5
- 25030088
-
20
Tayler instability in Protoneutron stars PI/4-405 - Bob Room
PI/4-405 - Bob Room
Perimeter Institute for Theoretical Physics
60Amplification of magnetic fields by differential rotation and feedback by magnetic instabilities is one of the main mechanisms for magnetizing a protoneutron star. I will discuss a recent revision of the Tayler instability of strong toroidal fields and its implications for the stably stratified interior of protoneutron stars. If time permits, I will briefly highlight new simulations quantifying the efficiency of the chiral dynamo instability.
Speaker: Valentin Skoutnev- 25030117
- 380501fb-7b32-40fc-987c-ae71886173ef
-
12:00 p.m.
Seminar PI/4-405 - Bob Room
PI/4-405 - Bob Room
Perimeter Institute for Theoretical Physics
60 -
1:00 p.m.
Lunch PI/4-405 - Bob Room
PI/4-405 - Bob Room
Perimeter Institute for Theoretical Physics
60 -
21
Relativistic Gas Accretion onto Supermassive Black Hole Binaries from Inspiral through Merger PI/4-405 - Bob Room
PI/4-405 - Bob Room
Perimeter Institute for Theoretical Physics
60Accreting supermassive black hole binaries are powerful multimessenger sources emitting both gravitational and electromagnetic (EM) radiation. Understanding the accretion dynamics of these systems and predicting their distinctive EM signals is crucial to informing and guiding upcoming efforts aimed at detecting gravitational waves produced by these binaries. To this end, accurate numerical modeling is required to describe both the spacetime and the magnetized gas around the black holes. In this talk, I will outline two key advancements in this field of research.
On the one hand, I will present a novel 3D general relativistic magnetohydrodynamics (GRMHD) framework that combines multiple numerical codes to simulate the inspiral and merger of supermassive black hole binaries starting from realistic initial data and running all the way through merger. Throughout the evolution, we adopt a simple but functional prescription to account for gas cooling through the emission of photons.
On the other hand, I will present the application of our new computational method to following the time evolution of a circular, equal-mass, non-spinning black hole binary of total mass ${M}$ for ${\sim\!200}$ orbits starting from a separation of ${20\,r_g\equiv 20\,M}$ and reaching the post-merger evolutionary stage of the system. Our simulation has confirmed the predictions of previous works about the early inspiral phase, but has also revealed phenomena specific to the late-inspiral and merger so far undocumented in the literature. Perhaps our most striking finding is that, although the accretion rate onto the black holes is approximately constant from ${\sim\!3000\,M}$ before merger onward, the EM luminosity undergoes a sharp increase around the time of merger. This effect is caused by the sudden lack of binary torque, which allows the gas in the immediate vicinity of the remnant to quickly fall in, thus compressing and heating up as it shocks. Secondly, the magnetic flux brought to the ${\sim\!0.68\text{-spinning}}$ merger remnant is able to drive a relativistic, Poynting-flux-dominated jet.
These dynamics could lead to potentially observable EM signals, supporting upcoming multimessenger observational campaigns.
Speaker: Lorenzo Ennoggi (Rochester Institute of Technology)- 25030144
- 9c63cc1a-fdcb-404f-af87-575abacbd0ff
-
22
Magnetar Formation via Accretion-Induced Collapse of White Dwarfs PI/4-405 - Bob Room
PI/4-405 - Bob Room
Perimeter Institute for Theoretical Physics
60We present the first two-dimensional axisymmetric Newtonian magnetohydrodynamic simulations of accretion-induced collapse (AIC) of rotating white dwarfs (WDs) with self-consistent initial magnetic progenitors and neutrino leakage. Our findings show that with initial surface magnetic field strength constrained by isolated WD observations, the protoneutron star can reach field strength consistent with magnetar observations. Our results suggest that single degenerate WDs can form magnetars via AIC.
Speaker: Mr Siu Hei Cheung (The Chinese University of Hong Kong)- 25030146
- 2d7cf0bf-05f8-4238-9bc9-49f4800adf4f
-
3:15 p.m.
Break PI/4-405 - Bob Room
PI/4-405 - Bob Room
Perimeter Institute for Theoretical Physics
60 -
Lightning Talk: Lightning Talks PI/4-405 - Bob Room
PI/4-405 - Bob Room
Perimeter Institute for Theoretical Physics
60-
23
Consequences of Low Resolution and High Initial Magnetic Fields in Binary Neutron Star Merger Simulations
Simulations of magnetized binary neutron star mergers often seed the interiors of the initial stars with unrealistically strong magnetic fields to overcome the suppression of small-scale turbulence by finite grid resolution and observe postmerger magnetic collimation and potential jet breakout. We present a curious numerical instability arising from low resolution (227 meters) and high initial dipolar fields ($E_{tot} = 5 \times 10^{49}$ ergs) observed when conducting BNS mergers in a full 3D domain. Initial poloidal structures of sufficient magnitude can linger within the merger remnant, even through the turbulent merger process. Differential rotation then winds these structures into two counterrotating torii separated by the x-y plane. Numerical diffusion inherent to the low resolution grid then causes counterrotating field lines to interact near the x-y plane, leading to spurious magnetic energy dissipation that feeds back into fluid motion. We discuss the consequences of this feedback, including a large circular drift of the merger remnant, and how increased resolution or grid symmetry can alleviate this issue.
Speaker: Mr Allen Wen (Rochester Institute of Technology)- 0842fc84-1b75-4632-b247-fe6e221fc455
- 25030145
-
24
General Relativistic Magnetohydrodynamic Simulations of Circumbinary Disk Accretion onto Unequal Mass Black Hole Binaries
Accreting supermassive binary black holes (SMBBHs), which are the expected outcome of galaxy mergers, are potential powerful multimessenger sources of gravitational waves (GWs) and electromagnetic (EM) radiation. The latter may be periodically modulated by an asymmetric density distribution in the circumbinary disk (CBD), typically referred to as the “lump”. Possible enhancement of this modulation is predicted to occur when considering unequal mass binaries. In that scenario the less massive black hole (often called the "secondary") is expected to consume a majority of the inflowing gas as it gets closer to the inner edge of the CBD possibly dominating the overall EM luminosity.
We perform the first set of full 3D general relativistic magnetohydrodynamic (GRMHD) simulations of astrophysically realistic unequal mass (q=1:2) black hole binaries, both spinning and non spinning, embedded in a CBD, adopting the IllinoisGRMHD code. We use relaxed initial data for the CBD retrieved from a previous long-term simulation, performed with the SphericalNR code, which employs curvilinear coordinates and a post-Newtonian (PN) metric with a cutout excising the central region containing the binary. We study the dependence of multiple diagnostics, including the mass accretion rate, the Poynting flux and the mass enclosed at different radii, on the spins of the black holes and their mass ratio. Additionally, we analyze the dynamics and structure of the minidisks surrounding each black hole and the evolution of the jets ejected by them.Speaker: Maria Chiara de Simone (Rochester Institute of Technology)- a0236cf9-cfb6-4589-8bf6-36ddec275afd
- 25030153
-
23
-
25
Panel Discussion #2 PI/4-405 - Bob Room
PI/4-405 - Bob Room
Perimeter Institute for Theoretical Physics
60- 25030138
- 04185fc1-dcd1-4b99-a939-f28ea8a46b3f
-
15
-
-
26
Numerical simulations of accreting neutron stars in a general relativistic framework. PI/4-405 - Bob Room
PI/4-405 - Bob Room
Perimeter Institute for Theoretical Physics
60Numerical modeling of accreting millisecond X-ray pulsars (AMXPs) allows us to understand the physical origin of different observational signatures detected from these systems. Since the birth sites of these signals are strongly influenced by the gravitational potential of the star, magnetohydrodynamic (MHD) simulations in full GR
(GRMHD) are essential to accurately capture space-time curvature effects and inherent variations in the X-ray spectra. In this talk, I will present results from 3D GRMHD simulations of accreting neutron stars with oblique magnetospheres. I will discuss the pulse profiles generated from the GRMHD simulations and their implications for mass-radius inference in the accreting sources. Apart from the surface features, AMXPs are also good candidates for studying neutron star jet formation mechanisms. Though there have been extensive investigations into black hole jets, neutron star jets remain highly unexplored. Our 2D axisymmetric study in the quiescent regime suggests that the thick disk collimates the initial open stellar flux, leading to jets like the Blandford-Znajek mechanism proposed for black holes. However, much remains to be done before we can draw a complete picture of jets launched from neutron stars. The global 3D GRMHD simulations of the accreting neutron stars allow us to explore the jet formation mechanisms in these systems in detail for the first time.Speaker: Pushpita Das- 25030100
- b91f68c4-baff-46a5-a7f1-2e1019872591
-
27
Transient Radio Emission of Quiescent Magnetars PI/4-405 - Bob Room
PI/4-405 - Bob Room
Perimeter Institute for Theoretical Physics
60Speaker: Alexander Philippov (UMD)- 25030101
- d9a752f8-135b-4eab-b3ad-4b386101cc9b
-
28
Hybrid Forcefree-(GR)MHD simulations of Magnetar Giant Flares PI/4-405 - Bob Room
PI/4-405 - Bob Room
Perimeter Institute for Theoretical Physics
60Neutron stars (NSs) represent exceptional astrophysical laboratories, exhibiting extreme magnetic fields and dynamic magnetospheres that drive diverse high-energy phenomena such as giant flares in magnetars. In this talk, I will discuss my research on driving giant flares from non-spinning neutron stars via twisting of magnetic field footpoints, simulated with my new hybrid forcefree-(general relativistic) magnetohydrodynamics code H-AMR. I will focus on the production and evolution of a hot inner magnetosphere (called the 'fireball') and a magnetized bubble, which is one of the leading progenitors of Fast Radio Bursts.
Speaker: Dr Koushik Chatterjee (University of Maryland)- 99a76648-374e-4883-955b-8bb359995656
- 25030147
-
29
Magnetic Field Evolution and Superconductivity of Pulsars PI/4-405 - Bob Room
PI/4-405 - Bob Room
Perimeter Institute for Theoretical Physics
60Radio pulsars display a range of puzzling long-term variability in their magnetospheric emission and spin-down. In this talk I will discuss the mechanisms for internal magnetic field evolution of pulsars, how it differs from the MHD of classical conducting fluids, and how the neutron star interior couples to the magnetosphere and its radiation.
Speaker: Dr Ashley Bransgrove (Princeton University, PCTS)- d9e78ad3-c789-4375-9dfa-b9eef0e0cd8c
- 25030148
-
10:30 a.m.
Break PI/4-405 - Bob Room
PI/4-405 - Bob Room
Perimeter Institute for Theoretical Physics
60 -
30
Radiation from fast magnetic dissipation around compact objects PI/4-405 - Bob Room
PI/4-405 - Bob Room
Perimeter Institute for Theoretical Physics
60Fast magnetic dissipation powers the bursting activity of isolated neutron stars, merging neutron stars, and flares from black holes. Four mechanisms of fast dissipation can operate in the magnetospheres of compact objects: Alfvenic turbulent cascade, magnetic reconnection, collision of Alfvén waves, and monsters shocks. Radiation produced by these dissipation modes is controlled by the compactness parameter. The main radiative output is usually in X-rays; in some cases, radio bursts are produced.
Speaker: Andrei Beloborodov (Columbia University)- 556f3cb7-a8f3-4654-ab81-792e8e9aefe0
- 25030173
-
31
GRMHD simulations of accretion disks: QPOs, truncated disks and QPOs from truncated disks PI/4-405 - Bob Room
PI/4-405 - Bob Room
Perimeter Institute for Theoretical Physics
60Black hole X-ray binaries (BHXRBs) and Active Galactic Nuclei (AGN)
transition through a series of accretion states in a well-defined order.
The accretion states, each associated with different luminosities,
spectral and variability characteristics, quasi periodic oscillations
(QPOs) and outflow properties, are thought to be triggered by physical
changes in the accretion disk around the central black hole.The
mechanisms behind state transitions, the geometry of transitional disks
and the physical mechanisms driving the emission characteristics we
observe remain highly debated.General relativistic magneto-hydrodynamic simulations (GRMHD) are
increasingly providing crucial insights into the accretion process, the
launch of outflows and the physical processes driving state transitions
in BHXRBs and AGN. Using GRMHD simulations conducted with the H-AMR code
I: 1) Discuss how high and low-frequency QPOs can be produced by a
highly tilted, geometrically thin accretion disk. 2) Present the first
GRMHD simulation showing the self-consistent formation of a truncated
accretion disk– a proposed disk model for the hard intermediate
accretion state, in which the accretion flow is thick and hot close to
the black hole, while the outer regions of the flow are thin and cool.
3) Describe how QPOs can be generated at the truncation radius (the
radius at which the disk transitions from thick to thin) in a truncated
accretion disk.Speaker: Gibwa Musoke (Canadian Institute for Theoretical Astrophysics)- 25030122
- dad36c0c-e004-4754-b2c7-48159eb7c446
-
32
Modeling X-ray emission in radiation-rich magnetar magnetospheres PI/4-405 - Bob Room
PI/4-405 - Bob Room
Perimeter Institute for Theoretical Physics
60Neutron star magnetospheres are a source of abundant X-ray activity. They have transients observed in different bands, like the fast radio burst (FRB) and associated hard X-ray flare from the Galactic magnetar SGR 1935+2154. We present global models for magnetar X-ray emission, including a landmark first-principle radiative particle-in-cell simulation of the twisted magnetar magnetosphere with the GPU-PIC code Entity. In one scenario, plasma particles accelerated by surface-motion-induced discharges interact resonantly with thermal background photons. Our GPU-accelerated particle-in-cell simulations track up-scattered high-energy photons that drive secondary pair production and ignite a magnetospheric circuit that persistently generates X-rays. We divulge the plasma properties of such a magnetospheric circuit, including densities and velocities, and give an outlook on alternative ignition scenarios for persistent magnetar X-ray emission.
Speaker: Jens Mahlmann (Dartmouth College)- 25030149
- 0e4f7042-17ac-4642-88ea-ec8f27d3e3d3
-
12:30 p.m.
Lunch PI/4-405 - Bob Room
PI/4-405 - Bob Room
Perimeter Institute for Theoretical Physics
60 -
33
Fast radio bursts as precursor radio emission from monster shocks PI/4-405 - Bob Room
PI/4-405 - Bob Room
Perimeter Institute for Theoretical Physics
60It has been proposed recently that the breaking of MHD waves in the inner
magnetosphere of strongly magnetized neutron stars can power different types of high-energy transients. We have studied the steepening and dissipation of a strongly magnetized fast magnetosonic wave propagating in a declining background magnetic field, by means of particle-in-cell simulations that encompass MHD scales. Our analysis confirms the formation of a monster shock as $B^2-E^2$ approaches zero, that dissipates about half of the fast magnetosonic wave energy, and reveals, for the first time, the generation of a high-frequency precursor wave at the monster shock, carrying a fraction of 0.001 of the total energy dissipated at the shock. The spectrum of the precursor wave exhibits several sharp harmonic peaks, with frequencies in the GHz band under conditions anticipated in magnetars. Such signals may appear as fast radio bursts.Speaker: Amir Levinson (Tel Aviv university)- 768987ab-e3f1-43fd-a5eb-acc2ee9bf942
- 25030151
-
34
Particle Acceleration in Magnetically-Dominated Turbulence PI/4-405 - Bob Room
PI/4-405 - Bob Room
Perimeter Institute for Theoretical Physics
60Building on recent advancements in understanding particle transport in magnetized media, we present a first-principles scaling law for the formation of non-thermal tails in particle spectra within mildly and strongly magnetized turbulent plasmas. This scaling is validated using results from kinetic Particle-In-Cell simulations, which show excellent agreement with our theoretical predictions. Finally, we discuss the astrophysical implications of these findings, particularly for the proton spectra in the coronae of supermassive black holes.
Speaker: Rostom Mbarek- 25030150
- af6d2a7e-90b6-4ea2-9678-e77237c8824c
-
2:45 p.m.
Break PI/4-405 - Bob Room
PI/4-405 - Bob Room
Perimeter Institute for Theoretical Physics
60 -
35
Panel Discussion #3 PI/4-405 - Bob Room
PI/4-405 - Bob Room
Perimeter Institute for Theoretical Physics
60- 0154ec6f-5c63-49da-9da3-cdc49ff3ada9
- 25030139
-
36
Closing Remarks PI/4-405 - Bob Room
PI/4-405 - Bob Room
Perimeter Institute for Theoretical Physics
60
-
26